Nanomedicine internalization and penetration: why should we use spheroids?
DOI:
https://doi.org/10.48797/sl.2022.12Keywords:
3D tumour spheroids, cancer, nanomedicine, tumour microenvironmentAbstract
Cancer is a leading cause of death worldwide and, despite medical advances in the development of new anticancer therapies, drug resistance, complicated side effects and poor efficacy remain a major public health problem. To breakdown the antisuppressive tumour strategies and to improve drug efficiency, nanomedicine is increasingly being used in anticancer therapy studies. Different in vitro experimental models and innovative approaches have been used in cancer research. In this aspect, 3D spheroids have become an attractive tool, overcoming many of the issues associated with using 2D models. Thus, in this review, an overview on 3D spheroids is provided, focusing on their use in the cancer field as a promising platform for nanomedicine studies. Thereafter, we highlight the main drug delivery systems currently developed and their applicability in 3D cell cultures.
References
Jing, X.; Yang, F.; Shao, C.; Wei, K.; Xie, M.; Shen, H.; Shu, Y. Role of Hypoxia in Cancer Therapy by Regulating the Tumor Microenvironment. Mol Cancer 2019, 18, 157, doi:10.1186/s12943-019-1089-9.
Nagai, H.; Kim, Y.H. Cancer Prevention from the Perspective of Global Cancer Burden Patterns. J Thorac Dis 2017, 9, 448–451, doi:10.21037/jtd.2017.02.75.
Ward, R.A.; Fawell, S.; Floc’h, N.; Flemington, V.; McKerrecher, D.; Smith, P.D. Challenges and Opportunities in Cancer Drug Resistance. Chem Rev 2021, 121, 3297–3351, doi:10.1021/acs.chemrev.0c00383.
World Health, O. WHO Report on Cancer: Setting Priorities, Investing Wisely and Providing Care for All; World Health Organization: Geneva, 2020; ISBN 9789240001299 9789240001305 (print version).
Öjlert, Å.K.; Halvorsen, A.R.; Nebdal, D.; Lund-Iversen, M.; Solberg, S.; Brustugun, O.T.; Lingjaerde, O.C.; Helland, Å. The Immune Microenvironment in Non-Small Cell Lung Cancer Is Predictive of Prognosis after Surgery. Mol Oncol 2019, 13, 1166–1179, doi:10.1002/1878-0261.12475.
Schabath, M.B.; Cote, M.L. Cancer Progress and Priorities: Lung Cancer. Cancer Epidemiol Biomarkers Prev 2019, 28, 1563–1579, doi:10.1158/1055-9965.EPI-19-0221.
Spiro, S.G.; Shah, P.L.; Rintoul, R.C.; George, J.; Janes, S.; Callister, M.; Novelli, M.; Shaw, P.; Kocjan, G.; Griffiths, C.; et al. Sequential Screening for Lung Cancer in a High-Risk Group: Randomised Controlled Trial: LungSEARCH: A Randomised Controlled Trial of Surveillance Using Sputum and Imaging for the EARly Detection of Lung Cancer in a High-Risk Group. Eur Respir J 2019, 54, 1900581, doi:10.1183/13993003.00581-2019.
van der Meel, R.; Sulheim, E.; Shi, Y.; Kiessling, F.; Mulder, W.J.M.; Lammers, T. Smart Cancer Nanomedicine. Nat Nanotechnol 2019, 14, 1007–1017, doi:10.1038/s41565-019-0567-y.
Shi, J.; Kantoff, P.W.; Wooster, R.; Farokhzad, O.C. Cancer Nanomedicine: Progress, Challenges and Opportunities. Nat Rev Cancer 2017, 17, 20–37, doi:10.1038/nrc.2016.108.
Bousbaa, H. Novel Anticancer Strategies. Pharmaceutics 2021, 13, doi:10.3390/pharmaceutics13020275.
Bala Tannan, N.; Manzari, M.T.; Herviou, L.; da Silva Ferreira, M.; Hagen, C.; Kiguchi, H.; Manova-Todorova, K.; Seshan, V.; de Stanchina, E.; Heller, D.A.; et al. Tumor-Targeted Nanoparticles Improve the Therapeutic Index of BCL2 and MCL1 Dual Inhibition. Blood 2021, 137, 2057–2069, doi:10.1182/blood.2020008017.
Mitchell, M.J.; Billingsley, M.M.; Haley, R.M.; Wechsler, M.E.; Peppas, N.A.; Langer, R. Engineering Precision Nanoparticles for Drug Delivery. Nat Rev Drug Discov 2021, 20, 101–124, doi:10.1038/s41573-020-0090-8.
Golombek, S.K.; May, J.N.; Theek, B.; Appold, L.; Drude, N.; Kiessling, F.; Lammers, T. Tumor Targeting via EPR: Strategies to Enhance Patient Responses. Adv Drug Deliv Rev 2018, 130, 17–38, doi:10.1016/j.addr.2018.07.007.
Martins, C.; Sousa, F.; Araújo, F.; Sarmento, B. Functionalizing PLGA and PLGA Derivatives for Drug Delivery and Tissue Regeneration Applications. Adv Healthc Mater 2018, 7, doi:10.1002/adhm.201701035.
Alexis, F.; Pridgen, E.; Molnar, L.K.; Farokhzad, O.C. Factors Affecting the Clearance and Biodistribution of Polymeric Nanoparticles. Mol Pharm 2008, 5, 505–515, doi:10.1021/mp800051m.
Mulvihill, J.J.; Cunnane, E.M.; Ross, A.M.; Duskey, J.T.; Tosi, G.; Grabrucker, A.M. Drug Delivery across the Blood-Brain Barrier: Recent Advances in the Use of Nanocarriers. Nanomedicine (Lond) 2020, 15, 205–214, doi:10.2217/nnm-2019-0367.
Nascimento, A. v; Singh, A.; Bousbaa, H.; Ferreira, D.; Sarmento, B.; Amiji, M.M. Combinatorial-Designed Epidermal Growth Factor Receptor-Targeted Chitosan Nanoparticles for Encapsulation and Delivery of Lipid-Modified Platinum Derivatives in Wild-Type and Resistant Non-Small-Cell Lung Cancer Cells. Mol Pharm 2015, 12, 4466–4477, doi:10.1021/acs.molpharmaceut.5b00642.
Fan, K.; Jia, X.; Zhou, M.; Wang, K.; Conde, J.; He, J.; Tian, J.; Yan, X. Ferritin Nanocarrier Traverses the Blood Brain Barrier and Kills Glioma. ACS Nano 2018, 12, 4105–4115, doi:10.1021/acsnano.7b06969.
Gothwal, A.; Kumar, H.; Nakhate, K.T.; Ajazuddin; Dutta, A.; Borah, A.; Gupta, U. Lactoferrin Coupled Lower Generation PAMAM Dendrimers for Brain Targeted Delivery of Memantine in Aluminum-Chloride-Induced Alzheimer’s Disease in Mice. Bioconjug Chem 2019, 30, 2573–2583, doi:10.1021/acs.bioconjchem.9b00505.
Martins, C.; Araújo, F.; Gomes, M.J.; Fernandes, C.; Nunes, R.; Li, W.; Santos, H.A.; Borges, F.; Sarmento, B. Using Microfluidic Platforms to Develop CNS-Targeted Polymeric Nanoparticles for HIV Therapy. Eur J Pharm Biopharm 2019, 138, 111–124, doi:10.1016/j.ejpb.2018.01.014.
Zhang, W.; Kohane, D.S. Keeping Nanomedicine on Target. Nano Lett 2021, 21, 3–5, doi:10.1021/acs.nanolett.0c04638.
van der Meel, R.; Lammers, T.; Hennink, W.E. Cancer Nanomedicines: Oversold or Underappreciated? Expert Opin Drug Deliv 2017, 14, 1–5, doi:10.1080/17425247.2017.1262346.
Gillet, J.P.; Varma, S.; Gottesman, M.M. The Clinical Relevance of Cancer Cell Lines. J Natl Cancer Inst 2013, 105, 452–458, doi:10.1093/jnci/djt007.
Katt, M.E.; Placone, A.L.; Wong, A.D.; Xu, Z.S.; Searson, P.C. In Vitro Tumor Models: Advantages, Disadvantages, Variables, and Selecting the Right Platform. Front Bioeng Biotechnol 2016, 4, 12, doi:10.3389/fbioe.2016.00012.
Hamilton, G.; Rath, B. Applicability of Tumor Spheroids for in Vitro Chemosensitivity Assays. Expert Opin Drug Metab Toxicol 2019, 15, 15–23, doi:10.1080/17425255.2019.1554055.
Alghuwainem, A.; Alshareeda, A.T.; Alsowayan, B. Scaffold-Free 3-D Cell Sheet Technique Bridges the Gap between 2-D Cell Culture and Animal Models. Int J Mol Sci 2019, 20, doi:10.3390/ijms20194926.
Carver, K.; Ming, X.; Juliano, R.L. Tumor Cell-Targeted Delivery of Nanoconjugated Oligonucleotides in Composite Spheroids. Nucleic Acid Ther 2014, 24, 413–419, doi:10.1089/nat.2014.0493.
Dong, Y.; Zheng, Q.; Wang, Z.; Lin, X.; You, Y.; Wu, S.; Wang, Y.; Hu, C.; Xie, X.; Chen, J.; et al. Higher Matrix Stiffness as an Independent Initiator Triggers Epithelial-Mesenchymal Transition and Facilitates HCC Metastasis. J Hematol Oncol 2019, 12, 112, doi:10.1186/s13045-019-0795-5.
Papaevangelou, E.; Boult, J.K.R.; Whitley, G.S.; Robinson, S.P.; Howe, F.A. Assessment of the Direct Effects of DDAH I on Tumour Angiogenesis in Vivo. Angiogenesis 2018, 21, 737–749, doi:10.1007/s10456-018-9617-6.
Luo, H.; Su, J.; Sun, R.; Sun, Y.; Wang, Y.; Dong, Y.; Shi, B.; Jiang, H.; Li, Z. Coexpression of IL7 and CCL21 Increases Efficacy of CAR-T Cells in Solid Tumors without Requiring Preconditioned Lymphodepletion. Clin Cancer Res 2020, 26, 5494–5505, doi:10.1158/1078-0432.Ccr-20-0777.
Rodrigues, J.; Heinrich, M.A.; Teixeira, L.M.; Prakash, J. 3D In Vitro Model (R)Evolution: Unveiling Tumor-Stroma Interactions. Trends Cancer 2021, 7, 249–264, doi:10.1016/j.trecan.2020.10.009.
Hou, X.; Du, C.; Lu, L.; Yuan, S.; Zhan, M.; You, P.; Du, H. Opportunities and Challenges of Patient-Derived Models in Cancer Research: Patient-Derived Xenografts, Patient-Derived Organoid and Patient-Derived Cells. World J Surg Oncol 2022, 20, 37, doi:10.1186/s12957-022-02510-8.
Ben-David, U.; Ha, G.; Tseng, Y.Y.; Greenwald, N.F.; Oh, C.; Shih, J.; McFarland, J.M.; Wong, B.; Boehm, J.S.; Beroukhim, R.; et al. Patient-Derived Xenografts Undergo Mouse-Specific Tumor Evolution. Nat Genet 2017, 49, 1567–1575, doi:10.1038/ng.3967.
Courau, T.; Bonnereau, J.; Chicoteau, J.; Bottois, H.; Remark, R.; Assante Miranda, L.; Toubert, A.; Blery, M.; Aparicio, T.; Allez, M.; et al. Cocultures of Human Colorectal Tumor Spheroids with Immune Cells Reveal the Therapeutic Potential of MICA/B and NKG2A Targeting for Cancer Treatment. J Immunother Cancer 2019, 7, 74, doi:10.1186/s40425-019-0553-9.
Tchoryk, A.; Taresco, V.; Argent, R.H.; Ashford, M.; Gellert, P.R.; Stolnik, S.; Grabowska, A.; Garnett, M.C. Penetration and Uptake of Nanoparticles in 3D Tumor Spheroids. Bioconjug Chem 2019, 30, 1371–1384, doi:10.1021/acs.bioconjchem.9b00136.
Mikhail, A.S.; Eetezadi, S.; Allen, C. Multicellular Tumor Spheroids for Evaluation of Cytotoxicity and Tumor Growth Inhibitory Effects of Nanomedicines in Vitro: A Comparison of Docetaxel-Loaded Block Copolymer Micelles and Taxotere®. PLoS One 2013, 8, e62630, doi:10.1371/journal.pone.0062630.
Vinci, M.; Gowan, S.; Boxall, F.; Patterson, L.; Zimmermann, M.; Court, W.; Lomas, C.; Mendiola, M.; Hardisson, D.; Eccles, S.A. Advances in Establishment and Analysis of Three-Dimensional Tumor Spheroid-Based Functional Assays for Target Validation and Drug Evaluation. BMC Biol 2012, 10, 29, doi:10.1186/1741-7007-10-29.
Balkwill, F.R.; Capasso, M.; Hagemann, T. The Tumor Microenvironment at a Glance. J Cell Sci 2012, 125, 5591–5596, doi:10.1242/jcs.116392.
Weaver, V.M.; Petersen, O.W.; Wang, F.; Larabell, C.A.; Briand, P.; Damsky, C.; Bissell, M.J. Reversion of the Malignant Phenotype of Human Breast Cells in Three-Dimensional Culture and in Vivo by Integrin Blocking Antibodies. J Cell Biol 1997, 137, 231–245, doi:10.1083/jcb.137.1.231.
Pistore, C.; Giannoni, E.; Colangelo, T.; Rizzo, F.; Magnani, E.; Muccillo, L.; Giurato, G.; Mancini, M.; Rizzo, S.; Riccardi, M.; et al. DNA Methylation Variations Are Required for Epithelial-to-Mesenchymal Transition Induced by Cancer-Associated Fibroblasts in Prostate Cancer Cells. Oncogene 2017, 36, 5551–5566, doi:10.1038/onc.2017.159.
Wei, L.; Ye, H.; Li, G.; Lu, Y.; Zhou, Q.; Zheng, S.; Lin, Q.; Liu, Y.; Li, Z.; Chen, R. Cancer-Associated Fibroblasts Promote Progression and Gemcitabine Resistance via the SDF-1/SATB-1 Pathway in Pancreatic Cancer. Cell Death Dis 2018, 9, 1065, doi:10.1038/s41419-018-1104-x.
Wang, L.; Cao, L.; Wang, H.; Liu, B.; Zhang, Q.; Meng, Z.; Wu, X.; Zhou, Q.; Xu, K. Cancer-Associated Fibroblasts Enhance Metastatic Potential of Lung Cancer Cells through IL-6/STAT3 Signaling Pathway. Oncotarget 2017, 8, 76116–76128, doi:10.18632/oncotarget.18814.
O’Connell, J.T.; Sugimoto, H.; Cooke, V.G.; MacDonald, B.A.; Mehta, A.I.; LeBleu, V.S.; Dewar, R.; Rocha, R.M.; Brentani, R.R.; Resnick, M.B.; et al. VEGF-A and Tenascin-C Produced by S100A4+ Stromal Cells Are Important for Metastatic Colonization. Proc Natl Acad Sci U S A 2011, 108, 16002–16007, doi:10.1073/pnas.1109493108.
Gaggioli, C.; Hooper, S.; Hidalgo-Carcedo, C.; Grosse, R.; Marshall, J.F.; Harrington, K.; Sahai, E. Fibroblast-Led Collective Invasion of Carcinoma Cells with Differing Roles for RhoGTPases in Leading and Following Cells. Nat Cell Biol 2007, 9, 1392–1400, doi:10.1038/ncb1658.
Martinez-Outschoorn, U.E.; Lisanti, M.P.; Sotgia, F. Catabolic Cancer-Associated Fibroblasts Transfer Energy and Biomass to Anabolic Cancer Cells, Fueling Tumor Growth. Semin Cancer Biol 2014, 25, 47–60, doi:10.1016/j.semcancer.2014.01.005.
Kim, J.H.; Oh, S.H.; Kim, E.J.; Park, S.J.; Hong, S.P.; Cheon, J.H.; Kim, T.I.; Kim, W.H. The Role of Myofibroblasts in Upregulation of S100A8 and S100A9 and the Differentiation of Myeloid Cells in the Colorectal Cancer Microenvironment. Biochem Biophys Res Commun 2012, 423, 60–66, doi:10.1016/j.bbrc.2012.05.081.
Salmon, H.; Franciszkiewicz, K.; Damotte, D.; Dieu-Nosjean, M.C.; Validire, P.; Trautmann, A.; Mami-Chouaib, F.; Donnadieu, E. Matrix Architecture Defines the Preferential Localization and Migration of T Cells into the Stroma of Human Lung Tumors. J Clin Invest 2012, 122, 899–910, doi:10.1172/jci45817.
Hinshaw, D.C.; Shevde, L.A. The Tumor Microenvironment Innately Modulates Cancer Progression. Cancer Res 2019, 79, 4557–4566, doi:10.1158/0008-5472.Can-18-3962.
Pinto, B.; Henriques, A.C.; Silva, P.M.A.; Bousbaa, H. Three-Dimensional Spheroids as In Vitro Preclinical Models for Cancer Research. Pharmaceutics 2020, 12, doi:10.3390/pharmaceutics12121186.
Boutilier, A.J.; Elsawa, S.F. Macrophage Polarization States in the Tumor Microenvironment. Int J Mol Sci 2021, 22, doi:10.3390/ijms22136995.
Lei, X.; Lei, Y.; Li, J.K.; Du, W.X.; Li, R.G.; Yang, J.; Li, J.; Li, F.; Tan, H.B. Immune Cells within the Tumor Microenvironment: Biological Functions and Roles in Cancer Immunotherapy. Cancer Lett 2020, 470, 126–133, doi:10.1016/j.canlet.2019.11.009.
Devalaraja, S.; To, T.K.J.; Folkert, I.W.; Natesan, R.; Alam, M.Z.; Li, M.; Tada, Y.; Budagyan, K.; Dang, M.T.; Zhai, L.; et al. Tumor-Derived Retinoic Acid Regulates Intratumoral Monocyte Differentiation to Promote Immune Suppression. Cell 2020, 180, 1098-1114.e16, doi:10.1016/j.cell.2020.02.042.
Sielska, M.; Przanowski, P.; Wylot, B.; Gabrusiewicz, K.; Maleszewska, M.; Kijewska, M.; Zawadzka, M.; Kucharska, J.; Vinnakota, K.; Kettenmann, H.; et al. Distinct Roles of CSF Family Cytokines in Macrophage Infiltration and Activation in Glioma Progression and Injury Response. J Pathol 2013, 230, 310–321, doi:10.1002/path.4192.
Ku, M.C.; Wolf, S.A.; Respondek, D.; Matyash, V.; Pohlmann, A.; Waiczies, S.; Waiczies, H.; Niendorf, T.; Synowitz, M.; Glass, R.; et al. GDNF Mediates Glioblastoma-Induced Microglia Attraction but Not Astrogliosis. Acta Neuropathol 2013, 125, 609–620, doi:10.1007/s00401-013-1079-8.
Larionova, I.; Kazakova, E.; Gerashchenko, T.; Kzhyshkowska, J. New Angiogenic Regulators Produced by TAMs: Perspective for Targeting Tumor Angiogenesis. Cancers (Basel) 2021, 13, doi:10.3390/cancers13133253.
Yamaguchi, T.; Fushida, S.; Yamamoto, Y.; Tsukada, T.; Kinoshita, J.; Oyama, K.; Miyashita, T.; Tajima, H.; Ninomiya, I.; Munesue, S.; et al. Tumor-Associated Macrophages of the M2 Phenotype Contribute to Progression in Gastric Cancer with Peritoneal Dissemination. Gastric Cancer 2016, 19, 1052–1065, doi:10.1007/s10120-015-0579-8.
Gao, L.; Wang, F.Q.; Li, H.M.; Yang, J.G.; Ren, J.G.; He, K.F.; Liu, B.; Zhang, W.; Zhao, Y.F. CCL2/EGF Positive Feedback Loop between Cancer Cells and Macrophages Promotes Cell Migration and Invasion in Head and Neck Squamous Cell Carcinoma. Oncotarget 2016, 7, 87037–87051, doi:10.18632/oncotarget.13523.
Zhang, D.; Qiu, X.; Li, J.; Zheng, S.; Li, L.; Zhao, H. TGF-β Secreted by Tumor-Associated Macrophages Promotes Proliferation and Invasion of Colorectal Cancer via MiR-34a-VEGF Axis. Cell Cycle 2018, 17, 2766–2778, doi:10.1080/15384101.2018.1556064.
Quail, D.F.; Joyce, J.A. Microenvironmental Regulation of Tumor Progression and Metastasis. Nat Med 2013, 19, 1423–1437, doi:10.1038/nm.3394.
Jinushi, M.; Baghdadi, M.; Chiba, S.; Yoshiyama, H. Regulation of Cancer Stem Cell Activities by Tumor-Associated Macrophages. Am J Cancer Res 2012, 2, 529–539.
Hanahan, D.; Weinberg, R.A. Hallmarks of Cancer: The next Generation. Cell 2011, 144, 646–674, doi:10.1016/j.cell.2011.02.013.
Folkman, J. Tumor Angiogenesis: Therapeutic Implications. N Engl J Med 1971, 285, 1182–1186, doi:10.1056/nejm197111182852108.
Belousov, A.; Titov, S.; Shved, N.; Garbuz, M.; Malykin, G.; Gulaia, V.; Kagansky, A.; Kumeiko, V. The Extracellular Matrix and Biocompatible Materials in Glioblastoma Treatment. Front Bioeng Biotechnol 2019, 7, 341, doi:10.3389/fbioe.2019.00341.
Zhu, T.S.; Costello, M.A.; Talsma, C.E.; Flack, C.G.; Crowley, J.G.; Hamm, L.L.; He, X.; Hervey-Jumper, S.L.; Heth, J.A.; Muraszko, K.M.; et al. Endothelial Cells Create a Stem Cell Niche in Glioblastoma by Providing NOTCH Ligands That Nurture Self-Renewal of Cancer Stem-like Cells. Cancer Res 2011, 71, 6061–6072, doi:10.1158/0008-5472.Can-10-4269.
McCoy, M.G.; Nyanyo, D.; Hung, C.K.; Goerger, J.P.; Zipfel, R.W.; Williams, R.M.; Nishimura, N.; Fischbach, C. Endothelial Cells Promote 3D Invasion of GBM by IL-8-Dependent Induction of Cancer Stem Cell Properties. Sci Rep 2019, 9, 9069, doi:10.1038/s41598-019-45535-y.
Gouarderes, S.; Mingotaud, A.F.; Vicendo, P.; Gibot, L. Vascular and Extracellular Matrix Remodeling by Physical Approaches to Improve Drug Delivery at the Tumor Site. Expert Opin Drug Deliv 2020, 17, 1703–1726, doi:10.1080/17425247.2020.1814735.
Prieto-Vila, M.; Takahashi, R.U.; Usuba, W.; Kohama, I.; Ochiya, T. Drug Resistance Driven by Cancer Stem Cells and Their Niche. Int J Mol Sci 2017, 18, doi:10.3390/ijms18122574.
Wang, Q.; He, Z.; Huang, M.; Liu, T.; Wang, Y.; Xu, H.; Duan, H.; Ma, P.; Zhang, L.; Zamvil, S.S.; et al. Vascular Niche IL-6 Induces Alternative Macrophage Activation in Glioblastoma through HIF-2α. Nat Commun 2018, 9, 559, doi:10.1038/s41467-018-03050-0.
Wang, H.; Zhou, H.; Xu, J.; Lu, Y.; Ji, X.; Yao, Y.; Chao, H.; Zhang, J.; Zhang, X.; Yao, S.; et al. Different T-Cell Subsets in Glioblastoma Multiforme and Targeted Immunotherapy. Cancer Lett 2021, 496, 134–143, doi:10.1016/j.canlet.2020.09.028.
de Gooijer, M.C.; Guillén Navarro, M.; Bernards, R.; Wurdinger, T.; van Tellingen, O. An Experimenter’s Guide to Glioblastoma Invasion Pathways. Trends Mol Med 2018, 24, 763–780, doi:10.1016/j.molmed.2018.07.003.
Naba, A.; Clauser, K.R.; Ding, H.; Whittaker, C.A.; Carr, S.A.; Hynes, R.O. The Extracellular Matrix: Tools and Insights for the “Omics” Era. Matrix Biol 2016, 49, 10–24, doi:10.1016/j.matbio.2015.06.003.
Brassart-Pasco, S.; Brézillon, S.; Brassart, B.; Ramont, L.; Oudart, J.B.; Monboisse, J.C. Tumor Microenvironment: Extracellular Matrix Alterations Influence Tumor Progression. Front Oncol 2020, 10, 397, doi:10.3389/fonc.2020.00397.
Levental, K.R.; Yu, H.; Kass, L.; Lakins, J.N.; Egeblad, M.; Erler, J.T.; Fong, S.F.; Csiszar, K.; Giaccia, A.; Weninger, W.; et al. Matrix Crosslinking Forces Tumor Progression by Enhancing Integrin Signaling. Cell 2009, 139, 891–906, doi:10.1016/j.cell.2009.10.027.
Xiao, Q.; Ge, G. Lysyl Oxidase, Extracellular Matrix Remodeling and Cancer Metastasis. Cancer Microenviron 2012, 5, 261–273, doi:10.1007/s12307-012-0105-z.
Henke, E.; Nandigama, R.; Ergün, S. Extracellular Matrix in the Tumor Microenvironment and Its Impact on Cancer Therapy. Front Mol Biosci 2019, 6, 160, doi:10.3389/fmolb.2019.00160.
Provenzano, P.P.; Inman, D.R.; Eliceiri, K.W.; Knittel, J.G.; Yan, L.; Rueden, C.T.; White, J.G.; Keely, P.J. Collagen Density Promotes Mammary Tumor Initiation and Progression. BMC Med 2008, 6, 11, doi:10.1186/1741-7015-6-11.
Riching, K.M.; Cox, B.L.; Salick, M.R.; Pehlke, C.; Riching, A.S.; Ponik, S.M.; Bass, B.R.; Crone, W.C.; Jiang, Y.; Weaver, A.M.; et al. 3D Collagen Alignment Limits Protrusions to Enhance Breast Cancer Cell Persistence. Biophys J 2014, 107, 2546–2558, doi:10.1016/j.bpj.2014.10.035.
Han, W.; Chen, S.; Yuan, W.; Fan, Q.; Tian, J.; Wang, X.; Chen, L.; Zhang, X.; Wei, W.; Liu, R.; et al. Oriented Collagen Fibers Direct Tumor Cell Intravasation. Proc Natl Acad Sci U S A 2016, 113, 11208–11213, doi:10.1073/pnas.1610347113.
Chia, J.; Kusuma, N.; Anderson, R.; Parker, B.; Bidwell, B.; Zamurs, L.; Nice, E.; Pouliot, N. Evidence for a Role of Tumor-Derived Laminin-511 in the Metastatic Progression of Breast Cancer. Am J Pathol 2007, 170, 2135–2148, doi:10.2353/ajpath.2007.060709.
Lin, T.C.; Yang, C.H.; Cheng, L.H.; Chang, W.T.; Lin, Y.R.; Cheng, H.C. Fibronectin in Cancer: Friend or Foe. Cells 2019, 9, doi:10.3390/cells9010027.
Fujita, M.; Ito-Fujita, Y.; Iyoda, T.; Sasada, M.; Okada, Y.; Ishibashi, K.; Osawa, T.; Kodama, H.; Fukai, F.; Suzuki, H. Peptide TNIIIA2 Derived from Tenascin-C Contributes to Malignant Progression in Colitis-Associated Colorectal Cancer via Β1-Integrin Activation in Fibroblasts. Int J Mol Sci 2019, 20, doi:10.3390/ijms20112752.
Wei, J.; Marisetty, A.; Schrand, B.; Gabrusiewicz, K.; Hashimoto, Y.; Ott, M.; Grami, Z.; Kong, L.Y.; Ling, X.; Caruso, H.; et al. Osteopontin Mediates Glioblastoma-Associated Macrophage Infiltration and Is a Potential Therapeutic Target. J Clin Invest 2019, 129, 137–149, doi:10.1172/jci121266.
Deligne, C.; Murdamoothoo, D.; Gammage, A.N.; Gschwandtner, M.; Erne, W.; Loustau, T.; Marzeda, A.M.; Carapito, R.; Paul, N.; Velazquez-Quesada, I.; et al. Matrix-Targeting Immunotherapy Controls Tumor Growth and Spread by Switching Macrophage Phenotype. Cancer Immunol Res 2020, 8, 368–382, doi:10.1158/2326-6066.Cir-19-0276.
Deligne, C.; Midwood, K.S. Macrophages and Extracellular Matrix in Breast Cancer: Partners in Crime or Protective Allies? Front Oncol 2021, 11, 620773, doi:10.3389/fonc.2021.620773.
Jensen, C.; Teng, Y. Is It Time to Start Transitioning From 2D to 3D Cell Culture? Front Mol Biosci 2020, 7, 33, doi:10.3389/fmolb.2020.00033.
Ishiguro, T.; Ohata, H.; Sato, A.; Yamawaki, K.; Enomoto, T.; Okamoto, K. Tumor-Derived Spheroids: Relevance to Cancer Stem Cells and Clinical Applications. Cancer Sci 2017, 108, 283–289, doi:10.1111/cas.13155.
Chaicharoenaudomrung, N.; Kunhorm, P.; Noisa, P. Three-Dimensional Cell Culture Systems as an in Vitro Platform for Cancer and Stem Cell Modeling. World J Stem Cells 2019, 11, 1065–1083, doi:10.4252/wjsc.v11.i12.1065.
Petrova, V.; Annicchiarico-Petruzzelli, M.; Melino, G.; Amelio, I. The Hypoxic Tumour Microenvironment. Oncogenesis 2018, 7, 10, doi:10.1038/s41389-017-0011-9.
Weiswald, L.B.; Bellet, D.; Dangles-Marie, V. Spherical Cancer Models in Tumor Biology. Neoplasia 2015, 17, 1–15, doi:10.1016/j.neo.2014.12.004.
Muguruma, M.; Teraoka, S.; Miyahara, K.; Ueda, A.; Asaoka, M.; Okazaki, M.; Kawate, T.; Kuroda, M.; Miyagi, Y.; Ishikawa, T. Differences in Drug Sensitivity between Two-Dimensional and Three-Dimensional Culture Systems in Triple-Negative Breast Cancer Cell Lines. Biochem Biophys Res Commun 2020, 533, 268–274, doi:10.1016/j.bbrc.2020.08.075.
Souza, A.G.; Silva, I.B.B.; Campos-Fernandez, E.; Barcelos, L.S.; Souza, J.B.; Marangoni, K.; Goulart, L.R.; Alonso-Goulart, V. Comparative Assay of 2D and 3D Cell Culture Models: Proliferation, Gene Expression and Anticancer Drug Response. Curr Pharm Des 2018, 24, 1689–1694, doi:10.2174/1381612824666180404152304.
Melissaridou, S.; Wiechec, E.; Magan, M.; Jain, M. v; Chung, M.K.; Farnebo, L.; Roberg, K. The Effect of 2D and 3D Cell Cultures on Treatment Response, EMT Profile and Stem Cell Features in Head and Neck Cancer. Cancer Cell Int 2019, 19, 16, doi:10.1186/s12935-019-0733-1.
Koch, J.; Mönch, D.; Maaß, A.; Gromoll, C.; Hehr, T.; Leibold, T.; Schlitt, H.J.; Dahlke, M.H.; Renner, P. Three Dimensional Cultivation Increases Chemo- and Radioresistance of Colorectal Cancer Cell Lines. PLoS One 2021, 16, e0244513, doi:10.1371/journal.pone.0244513.
Thakuri, P.S.; Liu, C.; Luker, G.D.; Tavana, H. Biomaterials-Based Approaches to Tumor Spheroid and Organoid Modeling. Adv Healthc Mater 2018, 7, e1700980, doi:10.1002/adhm.201700980.
Millard, M.; Yakavets, I.; Zorin, V.; Kulmukhamedova, A.; Marchal, S.; Bezdetnaya, L. Drug Delivery to Solid Tumors: The Predictive Value of the Multicellular Tumor Spheroid Model for Nanomedicine Screening. Int J Nanomedicine 2017, 12, 7993–8007, doi:10.2147/ijn.S146927.
Rodallec, A.; Sicard, G.; Giacometti, S.; Carré, M.; Pourroy, B.; Bouquet, F.; Savina, A.; Lacarelle, B.; Ciccolini, J.; Fanciullino, R. From 3D Spheroids to Tumor Bearing Mice: Efficacy and Distribution Studies of Trastuzumab-Docetaxel Immunoliposome in Breast Cancer. Int J Nanomedicine 2018, 13, 6677–6688, doi:10.2147/ijn.S179290.
Nunes, A.S.; Barros, A.S.; Costa, E.C.; Moreira, A.F.; Correia, I.J. 3D Tumor Spheroids as in Vitro Models to Mimic in Vivo Human Solid Tumors Resistance to Therapeutic Drugs. Biotechnol Bioeng 2019, 116, 206–226, doi:10.1002/bit.26845.
Gupta, P.; Miller, A.; Olayanju, A.; Madhuri, T.K.; Velliou, E. A Systematic Comparative Assessment of the Response of Ovarian Cancer Cells to the Chemotherapeutic Cisplatin in 3D Models of Various Structural and Biochemical Configurations-Does One Model Type Fit All? Cancers (Basel) 2022, 14, 1274, doi:10.3390/cancers14051274.
Huang, Z.; Yu, P.; Tang, J. Characterization of Triple-Negative Breast Cancer MDA-MB-231 Cell Spheroid Model. Onco Targets Ther 2020, 13, 5395–5405, doi:10.2147/OTT.S249756.
Matsumura, Y.; Maeda, H. A New Concept for Macromolecular Therapeutics in Cancer Chemotherapy: Mechanism of Tumoritropic Accumulation of Proteins and the Antitumor Agent Smancs. Cancer Res 1986, 46, 6387–6392.
Yong, T.; Zhang, X.; Bie, N.; Zhang, H.; Zhang, X.; Li, F.; Hakeem, A.; Hu, J.; Gan, L.; Santos, H.A.; et al. Tumor Exosome-Based Nanoparticles Are Efficient Drug Carriers for Chemotherapy. Nat Commun 2019, 10, 3838, doi:10.1038/s41467-019-11718-4.
Yakavets, I.; Lassalle, H.P.; Scheglmann, D.; Wiehe, A.; Zorin, V.; Bezdetnaya, L. Temoporfin-in-Cyclodextrin-in-Liposome-A New Approach for Anticancer Drug Delivery: The Optimization of Composition. Nanomaterials (Basel) 2018, 8, doi:10.3390/nano8100847.
Chaturvedi, V.K.; Singh, A.; Singh, V.K.; Singh, M.P. Cancer Nanotechnology: A New Revolution for Cancer Diagnosis and Therapy. Curr Drug Metab 2019, 20, 416–429, doi:10.2174/1389200219666180918111528.
Fernandez-Fernandez, A.; Manchanda, R.; McGoron, A.J. Theranostic Applications of Nanomaterials in Cancer: Drug Delivery, Image-Guided Therapy, and Multifunctional Platforms. Appl Biochem Biotechnol 2011, 165, 1628–1651, doi:10.1007/s12010-011-9383-z.
Terreno, E.; Uggeri, F.; Aime, S. Image Guided Therapy: The Advent of Theranostic Agents. J Control Release 2012, 161, 328–337, doi:10.1016/j.jconrel.2012.05.028.
Khosa, A.; Reddi, S.; Saha, R.N. Nanostructured Lipid Carriers for Site-Specific Drug Delivery. Biomed Pharmacother 2018, 103, 598–613, doi:10.1016/j.biopha.2018.04.055.
Pandey, P.; Dureja, H. Recent Patents on Polymeric Nanoparticles for Cancer Therapy. Recent Pat Nanotechnol 2018, 12, 155–169, doi:10.2174/1872210512666180327120648.
Leyva-Gómez, G.; Piñón-Segundo, E.; Mendoza-Muñoz, N.; Zambrano-Zaragoza, M.L.; Mendoza-Elvira, S.; Quintanar-Guerrero, D. Approaches in Polymeric Nanoparticles for Vaginal Drug Delivery: A Review of the State of the Art. Int J Mol Sci 2018, 19, doi:10.3390/ijms19061549.
Mehnert, W.; Mäder, K. Solid Lipid Nanoparticles: Production, Characterization and Applications. Adv Drug Deliv Rev 2001, 47, 165–196, doi:10.1016/s0169-409x(01)00105-3.
Aldawsari, H.M.; Singh, S. Rapid Microwave-Assisted Cisplatin-Loaded Solid Lipid Nanoparticles: Synthesis, Characterization and Anticancer Study. Nanomaterials (Basel) 2020, 10, doi:10.3390/nano10030510.
Zielińska, A.; Ferreira, N.R.; Durazzo, A.; Lucarini, M.; Cicero, N.; Mamouni, S.E.; Silva, A.M.; Nowak, I.; Santini, A.; Souto, E.B. Development and Optimization of Alpha-Pinene-Loaded Solid Lipid Nanoparticles (SLN) Using Experimental Factorial Design and Dispersion Analysis. Molecules 2019, 24, doi:10.3390/molecules24152683.
Wang, H.; Li, L.; Ye, J.; Wang, R.; Wang, R.; Hu, J.; Wang, Y.; Dong, W.; Xia, X.; Yang, Y.; et al. Improving the Oral Bioavailability of an Anti-Glioma Prodrug CAT3 Using Novel Solid Lipid Nanoparticles Containing Oleic Acid-CAT3 Conjugates. Pharmaceutics 2020, 12, doi:10.3390/pharmaceutics12020126.
Lee, M.K.; Lim, S.J.; Kim, C.K. Preparation, Characterization and in Vitro Cytotoxicity of Paclitaxel-Loaded Sterically Stabilized Solid Lipid Nanoparticles. Biomaterials 2007, 28, 2137–2146, doi:10.1016/j.biomaterials.2007.01.014.
Fathy Abd-Ellatef, G.E.; Gazzano, E.; Chirio, D.; Hamed, A.R.; Belisario, D.C.; Zuddas, C.; Peira, E.; Rolando, B.; Kopecka, J.; Assem Said Marie, M.; et al. Curcumin-Loaded Solid Lipid Nanoparticles Bypass P-Glycoprotein Mediated Doxorubicin Resistance in Triple Negative Breast Cancer Cells. Pharmaceutics 2020, 12, doi:10.3390/pharmaceutics12020096.
Senthil Kumar, C.; Thangam, R.; Mary, S.A.; Kannan, P.R.; Arun, G.; Madhan, B. Targeted Delivery and Apoptosis Induction of Trans-Resveratrol-Ferulic Acid Loaded Chitosan Coated Folic Acid Conjugate Solid Lipid Nanoparticles in Colon Cancer Cells. Carbohydr Polym 2020, 231, 115682, doi:10.1016/j.carbpol.2019.115682.
Granja, A.; Lima-Sousa, R.; Alves, C.G.; de Melo-Diogo, D.; Pinheiro, M.; Sousa, C.T.; Correia, I.J.; Reis, S. Mitoxantrone-Loaded Lipid Nanoparticles for Breast Cancer Therapy - Quality-by-Design Approach and Efficacy Assessment in 2D and 3D in Vitro Cancer Models. Int J Pharm 2021, 607, 121044, doi:10.1016/j.ijpharm.2021.121044.
Arduino, I.; Liu, Z.; Rahikkala, A.; Figueiredo, P.; Correia, A.; Cutrignelli, A.; Denora, N.; Santos, H.A. Preparation of Cetyl Palmitate-Based PEGylated Solid Lipid Nanoparticles by Microfluidic Technique. Acta Biomater 2021, 121, 566–578, doi:10.1016/j.actbio.2020.12.024.
Arduino, I.; Liu, Z.; Iacobazzi, R.M.; Lopedota, A.A.; Lopalco, A.; Cutrignelli, A.; Laquintana, V.; Porcelli, L.; Azzariti, A.; Franco, M.; et al. Microfluidic Preparation and in Vitro Evaluation of IRGD-Functionalized Solid Lipid Nanoparticles for Targeted Delivery of Paclitaxel to Tumor Cells. Int J Pharm 2021, 610, 121246, doi:10.1016/j.ijpharm.2021.121246.
Garanti, T.; Alhnan, M.A.; Wan, K.W. RGD-Decorated Solid Lipid Nanoparticles Enhance Tumor Targeting, Penetration and Anticancer Effect of Asiatic Acid. Nanomedicine (Lond) 2020, 15, 1567–1583, doi:10.2217/nnm-2020-0035.
Allen, T.M.; Cullis, P.R. Liposomal Drug Delivery Systems: From Concept to Clinical Applications. Adv Drug Deliv Rev 2013, 65, 36–48, doi:10.1016/j.addr.2012.09.037.
Lai, F.; Fadda, A.M.; Sinico, C. Liposomes for Brain Delivery. Expert Opin Drug Deliv 2013, 10, 1003–1022, doi:10.1517/17425247.2013.766714.
Alavi, M.; Hamidi, M. Passive and Active Targeting in Cancer Therapy by Liposomes and Lipid Nanoparticles. Drug Metab Pers Ther 2019, 34, doi:10.1515/dmpt-2018-0032.
Dutta, S.; Moses, J.A.; Anandharamakrishnan, C. Encapsulation of Nutraceutical Ingredients in Liposomes and Their Potential for Cancer Treatment. Nutr Cancer 2018, 70, 1184–1198, doi:10.1080/01635581.2018.1557212.
Okamoto, Y.; Taguchi, K.; Imoto, S.; Giam Chuang, V.T.; Yamasaki, K.; Otagiri, M. Cell Uptake and Anti-Tumor Effect of Liposomes Containing Encapsulated Paclitaxel-Bound Albumin against Breast Cancer Cells in 2D and 3D Cultured Models. Journal of Drug Delivery Science and Technology 2020, 55, 101381, doi:https://doi.org/10.1016/j.jddst.2019.101381.
d’Avanzo, N.; Torrieri, G.; Figueiredo, P.; Celia, C.; Paolino, D.; Correia, A.; Moslova, K.; Teesalu, T.; Fresta, M.; Santos, H.A. LinTT1 Peptide-Functionalized Liposomes for Targeted Breast Cancer Therapy. Int J Pharm 2021, 597, 120346, doi:10.1016/j.ijpharm.2021.120346.
Hwang, D.; Ramsey, J.D.; Kabanov, A. v Polymeric Micelles for the Delivery of Poorly Soluble Drugs: From Nanoformulation to Clinical Approval. Adv Drug Deliv Rev 2020, 156, 80–118, doi:10.1016/j.addr.2020.09.009.
Almeida, A.; Araújo, M.; Novoa-Carballal, R.; Andrade, F.; Gonçalves, H.; Reis, R.L.; Lúcio, M.; Schwartz Jr., S.; Sarmento, B. Novel Amphiphilic Chitosan Micelles as Carriers for Hydrophobic Anticancer Drugs. Mater Sci Eng C Mater Biol Appl 2020, 112, 110920, doi:10.1016/j.msec.2020.110920.
Barve, A.; Jain, A.; Liu, H.; Zhao, Z.; Cheng, K. Enzyme-Responsive Polymeric Micelles of Cabazitaxel for Prostate Cancer Targeted Therapy. Acta Biomater 2020, 113, 501–511, doi:10.1016/j.actbio.2020.06.019.
Chen, Q.; Liang, H.; Sun, Y.; Chen, Y.; He, W.; Fang, X.; Sha, X.; Li, J. A Carbohydrate Mimetic Peptide Modified Size-Shrinkable Micelle Nanocluster for Anti-Tumor Targeting and Penetrating Drug Delivery. Int J Nanomedicine 2019, 14, 7339–7352, doi:10.2147/ijn.S213455.
Yokoyama, M. Polymeric Micelles as Drug Carriers: Their Lights and Shadows. J Drug Target 2014, 22, 576–583, doi:10.3109/1061186x.2014.934688.
Yousefpour Marzbali, M.; Yari Khosroushahi, A. Polymeric Micelles as Mighty Nanocarriers for Cancer Gene Therapy: A Review. Cancer Chemother Pharmacol 2017, 79, 637–649, doi:10.1007/s00280-017-3273-1.
Du, A.W.; Lu, H.; Stenzel, M.H. Core-Cross-Linking Accelerates Antitumor Activities of Paclitaxel-Conjugate Micelles to Prostate Multicellular Tumor Spheroids: A Comparison of 2D and 3D Models. Biomacromolecules 2015, 16, 1470–1479, doi:10.1021/acs.biomac.5b00282.
Movassaghian, S.; Merkel, O.M.; Torchilin, V.P. Applications of Polymer Micelles for Imaging and Drug Delivery. Wiley Interdiscip Rev Nanomed Nanobiotechnol 2015, 7, 691–707, doi:10.1002/wnan.1332.
Tian, L.; Pei, R.; Zhong, L.; Ji, Y.; Zhou, D.; Zhou, S. Enhanced Targeting of 3D Pancreatic Cancer Spheroids by Aptamer-Conjugated Polymeric Micelles with Deep Tumor Penetration. Eur J Pharmacol 2021, 894, 173814, doi:10.1016/j.ejphar.2020.173814.
Yao, Q.; Choi, J.H.; Dai, Z.; Wang, J.; Kim, D.; Tang, X.; Zhu, L. Improving Tumor Specificity and Anticancer Activity of Dasatinib by Dual-Targeted Polymeric Micelles. ACS Appl Mater Interfaces 2017, 9, 36642–36654, doi:10.1021/acsami.7b12233.
Sharma, H.; Mishra, P.K.; Talegaonkar, S.; Vaidya, B. Metal Nanoparticles: A Theranostic Nanotool against Cancer. Drug Discov Today 2015, 20, 1143–1151, doi:10.1016/j.drudis.2015.05.009.
Abdal Dayem, A.; Hossain, M.K.; Lee, S.B.; Kim, K.; Saha, S.K.; Yang, G.M.; Choi, H.Y.; Cho, S.G. The Role of Reactive Oxygen Species (ROS) in the Biological Activities of Metallic Nanoparticles. Int J Mol Sci 2017, 18, doi:10.3390/ijms18010120.
Yu, Z.; Li, Q.; Wang, J.; Yu, Y.; Wang, Y.; Zhou, Q.; Li, P. Reactive Oxygen Species-Related Nanoparticle Toxicity in the Biomedical Field. Nanoscale Res Lett 2020, 15, 115, doi:10.1186/s11671-020-03344-7.
Jeon, M.; Halbert, M. v; Stephen, Z.R.; Zhang, M. Iron Oxide Nanoparticles as T(1) Contrast Agents for Magnetic Resonance Imaging: Fundamentals, Challenges, Applications, and Prospectives. Adv Mater 2021, 33, e1906539, doi:10.1002/adma.201906539.
Sujai, P.T.; Joseph, M.M.; Saranya, G.; Nair, J.B.; Murali, V.P.; Maiti, K.K. Surface Charge Modulates the Internalization vs. Penetration of Gold Nanoparticles: Comprehensive Scrutiny on Monolayer Cancer Cells, Multicellular Spheroids and Solid Tumors by SERS Modality. Nanoscale 2020, 12, 6971–6975, doi:10.1039/d0nr00809e.
Lu, H.; Su, J.; Mamdooh, R.; Li, Y.; Stenzel, M.H. Cellular Uptake of Gold Nanoparticles and Their Movement in 3D Multicellular Tumor Spheroids: Effect of Molecular Weight and Grafting Density of Poly(2-Hydroxyl Ethyl Acrylate). Macromol Biosci 2020, 20, e1900221, doi:10.1002/mabi.201900221.
Bugno, J.; Poellmann, M.J.; Sokolowski, K.; Hsu, H.J.; Kim, D.H.; Hong, S. Tumor Penetration of Sub-10 Nm Nanoparticles: Effect of Dendrimer Properties on Their Penetration in Multicellular Tumor Spheroids. Nanomedicine 2019, 21, 102059, doi:10.1016/j.nano.2019.102059.
Huang, K.; Ma, H.; Liu, J.; Huo, S.; Kumar, A.; Wei, T.; Zhang, X.; Jin, S.; Gan, Y.; Wang, P.C.; et al. Size-Dependent Localization and Penetration of Ultrasmall Gold Nanoparticles in Cancer Cells, Multicellular Spheroids, and Tumors in Vivo. ACS Nano 2012, 6, 4483–4493, doi:10.1021/nn301282m.
Doppalapudi, S.; Jain, A.; Domb, A.J.; Khan, W. Biodegradable Polymers for Targeted Delivery of Anti-Cancer Drugs. Expert Opin Drug Deliv 2016, 13, 891–909, doi:10.1517/17425247.2016.1156671.
Gagliardi, A.; Giuliano, E.; Venkateswararao, E.; Fresta, M.; Bulotta, S.; Awasthi, V.; Cosco, D. Biodegradable Polymeric Nanoparticles for Drug Delivery to Solid Tumors. Front Pharmacol 2021, 12, 601626, doi:10.3389/fphar.2021.601626.
Elbatanony, R.S.; Parvathaneni, V.; Kulkarni, N.S.; Shukla, S.K.; Chauhan, G.; Kunda, N.K.; Gupta, V. Afatinib-Loaded Inhalable PLGA Nanoparticles for Localized Therapy of Non-Small Cell Lung Cancer (NSCLC)-Development and in-Vitro Efficacy. Drug Deliv Transl Res 2021, 11, 927–943, doi:10.1007/s13346-020-00802-8.
Vaidya, B.; Parvathaneni, V.; Kulkarni, N.S.; Shukla, S.K.; Damon, J.K.; Sarode, A.; Kanabar, D.; Garcia, J. v; Mitragotri, S.; Muth, A.; et al. Cyclodextrin Modified Erlotinib Loaded PLGA Nanoparticles for Improved Therapeutic Efficacy against Non-Small Cell Lung Cancer. Int J Biol Macromol 2019, 122, 338–347, doi:10.1016/j.ijbiomac.2018.10.181.
Guo, Y.; Wang, L.; Lv, P.; Zhang, P. Transferrin-Conjugated Doxorubicin-Loaded Lipid-Coated Nanoparticles for the Targeting and Therapy of Lung Cancer. Oncol Lett 2015, 9, 1065–1072, doi:10.3892/ol.2014.2840.
Sen, R.; Ganguly, S.; Ganguly, S.; Debnath, M.C.; Chakraborty, S.; Mukherjee, B.; Chattopadhyay, D. Apigenin-Loaded PLGA-DMSA Nanoparticles: A Novel Strategy to Treat Melanoma Lung Metastasis. Mol Pharm 2021, 18, 1920–1938, doi:10.1021/acs.molpharmaceut.0c00977.
Svenson, S.; Tomalia, D.A. Dendrimers in Biomedical Applications--Reflections on the Field. Adv Drug Deliv Rev 2005, 57, 2106–2129, doi:10.1016/j.addr.2005.09.018.
Saluja, V.; Mishra, Y.; Mishra, V.; Giri, N.; Nayak, P. Dendrimers Based Cancer Nanotheranostics: An Overview. Int J Pharm 2021, 600, 120485, doi:10.1016/j.ijpharm.2021.120485.
Palmerston Mendes, L.; Pan, J.; Torchilin, V.P. Dendrimers as Nanocarriers for Nucleic Acid and Drug Delivery in Cancer Therapy. Molecules 2017, 22, doi:10.3390/molecules22091401.
Jain, K.; Kesharwani, P.; Gupta, U.; Jain, N.K. Dendrimer Toxicity: Let’s Meet the Challenge. Int J Pharm 2010, 394, 122–142, doi:10.1016/j.ijpharm.2010.04.027.
Mignani, S.; Rodrigues, J.; Tomas, H.; Zablocka, M.; Shi, X.; Caminade, A.M.; Majoral, J.P. Dendrimers in Combination with Natural Products and Analogues as Anti-Cancer Agents. Chem Soc Rev 2018, 47, 514–532, doi:10.1039/c7cs00550d.
Bugno, J.; Hsu, H.J.; Pearson, R.M.; Noh, H.; Hong, S. Size and Surface Charge of Engineered Poly(Amidoamine) Dendrimers Modulate Tumor Accumulation and Penetration: A Model Study Using Multicellular Tumor Spheroids. Mol Pharm 2016, 13, 2155–2163, doi:10.1021/acs.molpharmaceut.5b00946.
Rompicharla, S.V.K.; Kumari, P.; Bhatt, H.; Ghosh, B.; Biswas, S. Biotin Functionalized PEGylated Poly(Amidoamine) Dendrimer Conjugate for Active Targeting of Paclitaxel in Cancer. Int J Pharm 2019, 557, 329–341, doi:10.1016/j.ijpharm.2018.12.069.
Moço, P.D.; Aharony, N.; Kamen, A. Adeno-Associated Viral Vectors for Homology-Directed Generation of CAR-T Cells. Biotechnol J 2020, 15, e1900286, doi:10.1002/biot.201900286.
Sandro, Q.; Relizani, K.; Benchaouir, R. AAV Production Using Baculovirus Expression Vector System. Methods Mol Biol 2019, 1937, 91–99, doi:10.1007/978-1-4939-9065-8_5.
Luo, J.; Luo, Y.; Sun, J.; Zhou, Y.; Zhang, Y.; Yang, X. Adeno-Associated Virus-Mediated Cancer Gene Therapy: Current Status. Cancer Lett 2015, 356, 347–356, doi:10.1016/j.canlet.2014.10.045.
Chandler, R.J.; Sands, M.S.; Venditti, C.P. Recombinant Adeno-Associated Viral Integration and Genotoxicity: Insights from Animal Models. Hum Gene Ther 2017, 28, 314–322, doi:10.1089/hum.2017.009.
Yanamandra, N.; Kondraganti, S.; Gondi, C.S.; Gujrati, M.; Olivero, W.C.; Dinh, D.H.; Rao, J.S. Recombinant Adeno-Associated Virus (RAAV) Expressing TFPI-2 Inhibits Invasion, Angiogenesis and Tumor Growth in a Human Glioblastoma Cell Line. Int J Cancer 2005, 115, 998–1005, doi:10.1002/ijc.20965.
Thorsen, F.; Afione, S.; Huszthy, P.C.; Tysnes, B.B.; Svendsen, A.; Bjerkvig, R.; Kotin, R.M.; Lønning, P.E.; Hoover, F. Adeno-Associated Virus (AAV) Serotypes 2, 4 and 5 Display Similar Transduction Profiles and Penetrate Solid Tumor Tissue in Models of Human Glioma. J Gene Med 2006, 8, 1131–1140, doi:10.1002/jgm.939.
Lee, S.; Ahn, H.J. Anti-EpCAM-Conjugated Adeno-Associated Virus Serotype 2 for Systemic Delivery of EGFR ShRNA: Its Retargeting and Antitumor Effects on OVCAR3 Ovarian Cancer in Vivo. Acta Biomater 2019, 91, 258–269, doi:10.1016/j.actbio.2019.04.044.
Oliveira, M.S.; Aryasomayajula, B.; Pattni, B.; Mussi, S. v; Ferreira, L.A.M.; Torchilin, V.P. Solid Lipid Nanoparticles Co-Loaded with Doxorubicin and α-Tocopherol Succinate Are Effective against Drug-Resistant Cancer Cells in Monolayer and 3-D Spheroid Cancer Cell Models. Int J Pharm 2016, 512, 292–300, doi:10.1016/j.ijpharm.2016.08.049.
Leiva, M.C.; Ortiz, R.; Contreras-Cáceres, R.; Perazzoli, G.; Mayevych, I.; López-Romero, J.M.; Sarabia, F.; Baeyens, J.M.; Melguizo, C.; Prados, J. Tripalmitin Nanoparticle Formulations Significantly Enhance Paclitaxel Antitumor Activity against Breast and Lung Cancer Cells in Vitro. Sci Rep 2017, 7, 13506, doi:10.1038/s41598-017-13816-z.
Dastidar, D.G.; Das, A.; Datta, S.; Ghosh, S.; Pal, M.; Thakur, N.S.; Banerjee, U.C.; Chakrabarti, G. Paclitaxel-Encapsulated Core-Shell Nanoparticle of Cetyl Alcohol for Active Targeted Delivery through Oral Route. Nanomedicine (Lond) 2019, 14, 2121–2150, doi:10.2217/nnm-2018-0419.
Hinger, D.; Navarro, F.; Käch, A.; Thomann, J.S.; Mittler, F.; Couffin, A.C.; Maake, C. Photoinduced Effects of M-Tetrahydroxyphenylchlorin Loaded Lipid Nanoemulsions on Multicellular Tumor Spheroids. J Nanobiotechnology 2016, 14, 68, doi:10.1186/s12951-016-0221-x.
Lukowski, J.K.; Weaver, E.M.; Hummon, A.B. Analyzing Liposomal Drug Delivery Systems in Three-Dimensional Cell Culture Models Using MALDI Imaging Mass Spectrometry. Anal Chem 2017, 89, 8453–8458, doi:10.1021/acs.analchem.7b02006.
Parvathaneni, V.; Kulkarni, N.S.; Shukla, S.K.; Farrales, P.T.; Kunda, N.K.; Muth, A.; Gupta, V. Systematic Development and Optimization of Inhalable Pirfenidone Liposomes for Non-Small Cell Lung Cancer Treatment. Pharmaceutics 2020, 12, doi:10.3390/pharmaceutics12030206.
Shukla, S.K.; Kulkarni, N.S.; Chan, A.; Parvathaneni, V.; Farrales, P.; Muth, A.; Gupta, V. Metformin-Encapsulated Liposome Delivery System: An Effective Treatment Approach against Breast Cancer. Pharmaceutics 2019, 11, doi:10.3390/pharmaceutics11110559.
Riaz, M.K.; Zhang, X.; Wong, K.H.; Chen, H.; Liu, Q.; Chen, X.; Zhang, G.; Lu, A.; Yang, Z. Pulmonary Delivery of Transferrin Receptors Targeting Peptide Surface-Functionalized Liposomes Augments the Chemotherapeutic Effect of Quercetin in Lung Cancer Therapy. Int J Nanomedicine 2019, 14, 2879–2902, doi:10.2147/ijn.S192219.
Kumari, P.; Rompicharla, S.V.K.; Muddineti, O.S.; Ghosh, B.; Biswas, S. Transferrin-Anchored Poly(Lactide) Based Micelles to Improve Anticancer Activity of Curcumin in Hepatic and Cervical Cancer Cell Monolayers and 3D Spheroids. Int J Biol Macromol 2018, 116, 1196–1213, doi:10.1016/j.ijbiomac.2018.05.040.
Sarisozen, C.; Abouzeid, A.H.; Torchilin, V.P. The Effect of Co-Delivery of Paclitaxel and Curcumin by Transferrin-Targeted PEG-PE-Based Mixed Micelles on Resistant Ovarian Cancer in 3-D Spheroids and in Vivo Tumors. Eur J Pharm Biopharm 2014, 88, 539–550, doi:10.1016/j.ejpb.2014.07.001.
Guo, X.; Wu, Z.; Li, W.; Wang, Z.; Li, Q.; Kong, F.; Zhang, H.; Zhu, X.; Du, Y.P.; Jin, Y.; et al. Appropriate Size of Magnetic Nanoparticles for Various Bioapplications in Cancer Diagnostics and Therapy. ACS Appl Mater Interfaces 2016, 8, 3092–3106, doi:10.1021/acsami.5b10352.
Huo, S.; Ma, H.; Huang, K.; Liu, J.; Wei, T.; Jin, S.; Zhang, J.; He, S.; Liang, X.-J. Superior Penetration and Retention Behavior of 50 Nm Gold Nanoparticles in Tumors. 2013, 73, 319–330, doi:10.1158/0008-5472.CAN-12-2071 %J Cancer Research.
Becker, A.; Lehrich, T.; Kalies, S.; Heisterkamp, A.; Ngezahayo, A. Parameters for Optoperforation-Induced Killing of Cancer Cells Using Gold Nanoparticles Functionalized With the C-Terminal Fragment of Clostridium Perfringens Enterotoxin. Int J Mol Sci 2019, 20, doi:10.3390/ijms20174248.
Katifelis, H.; Lyberopoulou, A.; Mukha, I.; Vityuk, N.; Grodzyuk, G.; Theodoropoulos, G.E.; Efstathopoulos, E.P.; Gazouli, M. Ag/Au Bimetallic Nanoparticles Induce Apoptosis in Human Cancer Cell Lines via P53, CASPASE-3 and BAX/BCL-2 Pathways. Artif Cells Nanomed Biotechnol 2018, 46, S389-s398, doi:10.1080/21691401.2018.1495645.
Li, H.; Li, Y.; Xiang, J.; Yang, X.; Li, C.; Liu, C.; Zhao, Q.; Zhou, L.; Gong, P.; Huang, J. Intelligent Bimetallic Nanoagents as Reactive Oxygen Species Initiator System for Effective Combination Phototherapy. 2020, 8, doi:10.3389/fbioe.2020.00423.
Zhong, Y.; Su, T.; Shi, Q.; Feng, Y.; Tao, Z.; Huang, Q.; Li, L.; Hu, L.; Li, S.; Tan, H.; et al. Co-Administration Of IRGD Enhances Tumor-Targeted Delivery And Anti-Tumor Effects Of Paclitaxel-Loaded PLGA Nanoparticles For Colorectal Cancer Treatment. Int J Nanomedicine 2019, 14, 8543–8560, doi:10.2147/ijn.S219820.
Younis, M.; Faming, W.; Hongyan, Z.; Mengmeng, T.; Hang, S.; Liudi, Y. Iguratimod Encapsulated PLGA-NPs Improves Therapeutic Outcome in Glioma, Glioma Stem-like Cells and Temozolomide Resistant Glioma Cells. Nanomedicine 2019, 22, 102101, doi:10.1016/j.nano.2019.102101.
Lazzari, G.; Vinciguerra, D.; Balasso, A.; Nicolas, V.; Goudin, N.; Garfa-Traore, M.; Fehér, A.; Dinnyés, A.; Nicolas, J.; Couvreur, P.; et al. Light Sheet Fluorescence Microscopy versus Confocal Microscopy: In Quest of a Suitable Tool to Assess Drug and Nanomedicine Penetration into Multicellular Tumor Spheroids. Eur J Pharm Biopharm 2019, 142, 195–203, doi:10.1016/j.ejpb.2019.06.019.
Al-Jamal, K.T.; Al-Jamal, W.T.; Wang, J.T.; Rubio, N.; Buddle, J.; Gathercole, D.; Zloh, M.; Kostarelos, K. Cationic Poly-L-Lysine Dendrimer Complexes Doxorubicin and Delays Tumor Growth in Vitro and in Vivo. ACS Nano 2013, 7, 1905–1917, doi:10.1021/nn305860k.
Cui, X.; Ma, C.; Vasudevaraja, V.; Serrano, J.; Tong, J.; Peng, Y.; Delorenzo, M.; Shen, G.; Frenster, J.; Morales, R.T.; et al. Dissecting the Immunosuppressive Tumor Microenvironments in Glioblastoma-on-a-Chip for Optimized PD-1 Immunotherapy. Elife 2020, 9, doi:10.7554/eLife.52253.
Kwak, T.J.; Lee, E. In Vitro Modeling of Solid Tumor Interactions with Perfused Blood Vessels. Sci Rep 2020, 10, 20142, doi:10.1038/s41598-020-77180-1.
Peirsman, A.; Blondeel, E.; Ahmed, T.; Anckaert, J.; Audenaert, D.; Boterberg, T.; Buzas, K.; Carragher, N.; Castellani, G.; Castro, F.; et al. MISpheroID: A Knowledgebase and Transparency Tool for Minimum Information in Spheroid Identity. Nature Methods 2021, 18, 1294–1303, doi:10.1038/s41592-021-01291-4.
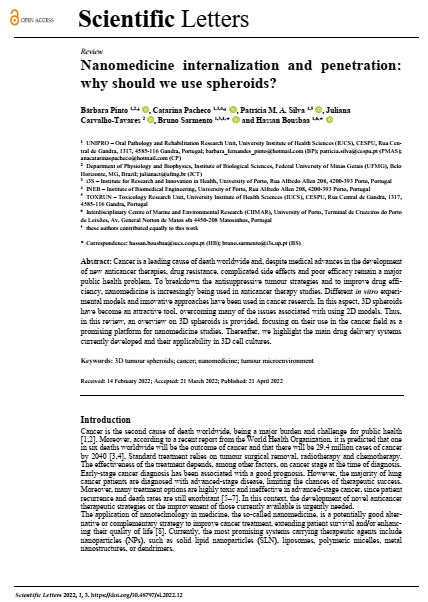
Downloads
Published
How to Cite
Issue
Section
License
Copyright (c) 2022 Bárbara Pinto, Catarina Pacheco, Patrícia Silva, Juliana Carvalho-Tavares , Bruno Sarmento, Hassan Bousbaa

This work is licensed under a Creative Commons Attribution 4.0 International License.
In Scientific Letters, articles are published under a CC-BY license (Creative Commons Attribution 4.0 International License), the most open license available. The users can share (copy and redistribute the material in any medium or format) and adapt (remix, transform, and build upon the material for any purpose, even commercially), as long as they give appropriate credit, provide a link to the license, and indicate if changes were made (read the full text of the license terms and conditions of use).
The author is the owner of the copyright.